
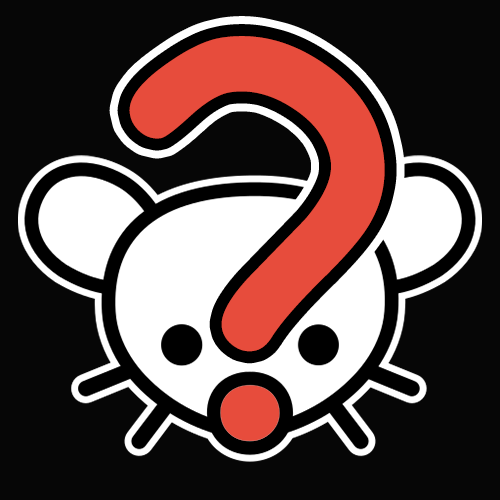
Not exactly, you can read piss shit fuck, however other words like maybe removed, removed, removed, removed may be censored
Edit: didn’t think this one through lmao
W hor e
Bi tch
G**k
N**er
Not exactly, you can read piss shit fuck, however other words like maybe removed, removed, removed, removed may be censored
Edit: didn’t think this one through lmao
W hor e
Bi tch
G**k
N**er
Argentina’s been gone for years
What is the cambridge 6 affair
OP if you’re serious about this email me at entrapment@fbi.gov
Spoiled vote
It’s bread stapled to a tree
I think you might be underestimating these guys a little, however skepticism is a part of science.
Replication experiments are being done right now so we’ll see what happens, but I’m sure they have adjusted for that
This article skips over a few points, heres the paper:
So your idea seems plausible at first, but more information leads to the proposed photo molecular effect:
Illumination of hydrogel under solar or visible-spectrum light-emitting diode leads to evaporation rates exceeding the thermal evaporation limit, even in hydrogels without additional absorbers.
Also the absorption of both the water and gel are negligible
I think the author’s theory about the photo molecular effect are right!
Basically they say that the photons cleave water molecules from the surface of the hydrogels.
At first i was not convinced but then i read the abstract of the journal article (its not on scihub yet) and it changed my mind, the news article skipped over a few points:
Not really, every bullet fired in ukraine weakens russia, an invading country and therefore it hurts soldiers.
The war in palestine kills innocent civilians and children
Ninja 400 for 6000 and that’s basically the honda civic of motorcycles. Very reliable even after being crashed multiple times
Why do cops need automatic weapons?
Egypt already has syrian refugees and subsaharan refugees in addition to the recent influx because of the sudanese civil war.
Either way, the current regime violently overthrew, imprisoned, tortured and eventually killed the democratically elected previous regime which has ties and many many sympathizers in Gaza. Meaning that the cocksucking son of a removed sisi will never let them in, because he’s afraid of another revolution
Plus leaked talks from Mubarak (two egyptian presidents ago) literally have bibi pointing at gaza and saying i want to move them elsewhere and pointed at sinai. If Palestinians go to sinai they are never coming home.
Not to mention that ISIS is in sinai…
I mean… This is a known fact. This is what tipped these idiots off
I only learned about this a couple days ago, but unfortunately delayed choice experiments do not prove retro causality.
It’s very disappointing, this guy also explains it on a physics forum, this anseer made sense to me but there are more in the thread:
This experiment implies retrocausality if you assume a classical way of thinking. The point of these experiments is to demonstrate that either retrocausality is real or the physics of the world are not precisely classical. The QM community, of course, would prefer to argue that their causal model is better than accepting retrocausality.
The tradeoff is interesting. Classical mechanics ensures every object has a simple state. A photon either went through slit A or slit B. However, to make this model describe the results of this real experiment, we need retrocausality. Quantum mechanics, on the other hand, gives each object a much more complicated concept of state. In QM, we assume a much weaker assumption about how the world works. We assume that the world can be modeled using linear operators. In the quantum way of thinking, photon goes through all paths at once (through both slit A and slit B), and has to have a complicated enough state to deal with that. We add the results of these two possible paths (something we can do because linear operators have a property called superposition).
In this QM interpretation, where the photon goes through slit A and slit B, we have to grapple with the intuition that most objects we know of go through one slit or another. It is the interpretations of QM that grapple with this, the most common being the Copenhagen Interpretation. The idea here is that if you design a sensor to react in a way which becomes increasingly classical after a detection (i.e. after the detection occurs, its state is well described classically), you have a “measurement.” The interpretations explain exactly what this measurement means. You and I are both much more comfortable with thinking classically, so the sooner we can get into thinking in terms of measurements of the quantum system, the sooner we can get back to handling the rest of the system (like the data collection computer) in a classical way!
I mention there are multiple interpretations. They all yield the same results of the measurements, statistically, but they all justify it differently. Each has its own quirks. Copenhagen has a random draw associated with measurement, which is easy to understand but suggests the measurement “did” something important. Others, like Pilot Wave avoid this random draw, but bring in other fun quirks. Copenhagen is the most popular, so I’m using that.
So in the QM world, the detectors on the bottom do not tell you what path the photon took. That would be meaningless, as the photon took all paths. What they do provide is a measurement that is heavily correlated to path information. If the system were thought of as a classical system, they would provide you which path the photon took, but we’re not thinking of it that way. It just provided a measurement providing path information.
Likewise, the gridded detector at the top does not tell you where the photon struck. That would be meaningless, at the photon “struck” everywhere. What it provides is a measurement that is related to the photons evolution.
Neither of these sensors provide anything unusual on their own. If you looked at the top part of the circuit, you could handwave away the quantumness and say that it told you where the photon struck classically. If you looked at the bottom part of the circuit, you could handwave away the quantumness and say it told you which path the photon took.
The oddity comes when you try to combine the measurements. Now quantum mechanics says this is two measurements of a singe photon with its complex state that took all paths. Now we can do a statistical correlation of them. This is where it gets interesting because the information here is not which-path, but a measurement related to paths. If the photon is detected by one of the sensors which provided path information in the classical sense, and we look at only those measurements taken by the grid, we see no interference pattern. The result of integrating all paths that lead the photon to a path-sensing sensor yields those measurements on the top sensor which are consistent with the photon taking one one path or the other.
If the photon is detected by the “path erasing” sensor in a classical sense, and we select only the grid detections associated with those, we see the interference patterns associated with the double slit. This is because, if we integrate all paths that lead the photon to the path-erasing sensor, they yield measurements on the top sensor that have fringes.
So what does this all mean? Well, it means the QM scientists came up with a complicated set of equations which modeled this one experiment better than classical mechanics did, without having to resort to retrocausality. Whooptie-doo. However, what is more interesting is to realize that this experiment is just one of many. Indeed, there are many quantum erasers experiments. If you are trying to model their results with classical mechanics, you have to come up with a retrocausality structure to explain each and every one of them. This leads to a long string of “what if I do this…” “what if I do that…” which exhausts time and resources! What if Bill and Ted come back from the future and tweak the path of my photon 29 years after they went to hell? The reformulation of classical mechanics to support this retrocausality is daunting!
The QM argument is that, rather than having to model everything with some retrocausality variant of classical mechanics, you can treat the “quantum” part of the system with one set of rules, and treat the entire rest of the world as a causal classical system. Now all of those ugly “What if” questions are answerable with “It will be the same as-if the rest (non-quantum) of the system was classical… go calculate what happens classically.” These more difficult equations and strange quantum concepts let us scope down the part of the system that is complicated (the one with all the beam splitters and brifringic crystals). And then, when Death comes to ask for best 5 out of 7, you know the odds, because the strange quantum behavior has been simplified into a random interaction with the larger classical system.
Deschutes County District Attorney Steve Gunnels said investigators had recovered journal entries with Garner’s belongings that indicated he was not planning to target specific people, but had a “generalized anger.” Court charging documents list potential victims in the case as “spectator” and “belayer.”
Cops claim that this is what the journal talks about, seems to be true. However, I’m sure the actual contents are out there somewhere or will be publicized during the trial if there is one
Alleged attemped mass murderer, innocent until proven guilty. You should not immediately jump to conclusions with insufficient evidence.
Allegations like these are enough to destroy a person’s life because of the fact that people always assume guilt.
Well it’s not mathematically possible
The formula is p/(2^n)
P would be the number of people you start with, and n is the number of games.
If you start with the population of the US, 350 million people, you can only do this for about 28 matches before you run out of people.
Infinity for lemmy / eternity for lemmy